Published on Nature Communications the work led by Samuele Ronchini, PhD student at GSSI and INFN associate, together with INAF, which sheds new light on cooling processes happening in jets of Gamma-Ray Bursts.
What are the processes that determine the cooling of relativistic particles in gamma-ray bursts producing the rapid flux decrease following the initial explosion? A convincing answer to this question comes from the work of a group of researchers working at the Gran Sasso Science Institute (GSSI), in collaboration with colleagues from the National Institute of Astrophysics (INAF). The researchers discover a relation shared by several gamma-ray bursts (GRBs) during a phase of rapid flux decrease, called “steep decay” phase. The uniqueness of this relation pushed the group to search for a universal process that could give a correct interpretation of the observations. The study has been published today in the journal Nature Communications.
Gamma-ray bursts are considered to be among the most catastrophic and energetic events in the Universe since they can release in few fractions of seconds the entire energy emitted by a star like the Sun throughout its entire life.
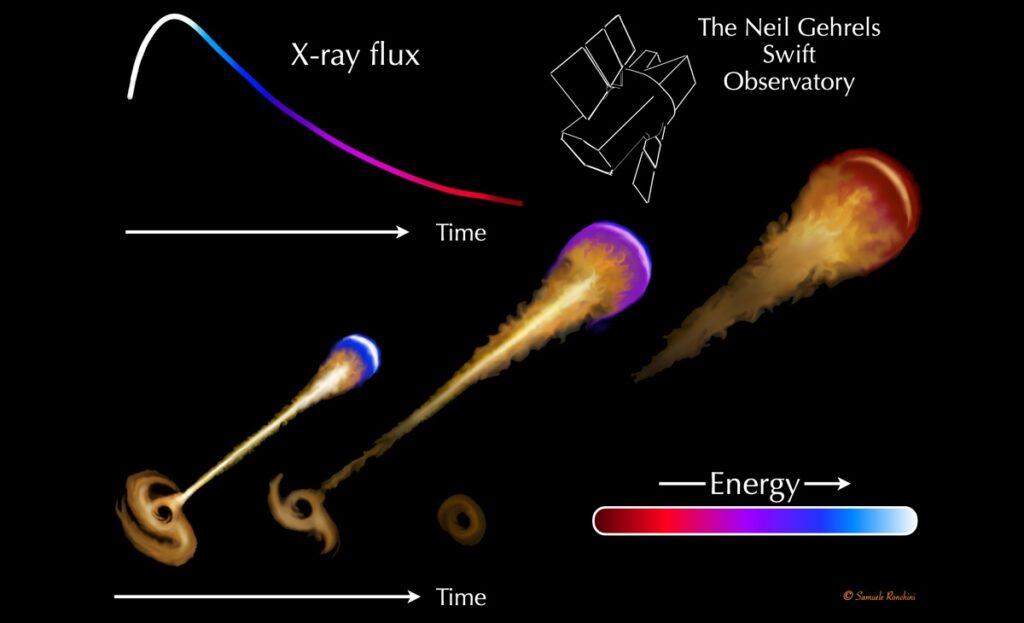
The discovery of supernovae and gravitational waves associated with GRBs confirmed that some of these phenomena are the product of the collapse of a massive star, and others of the merger of two neutron stars. Known since the 1960s, their nature remained debated until the advent of the Neil Gehrels “Swift” Observatory, a telescope in orbit since 2004 dedicated to the detection, localization and characterization of GRBs. After years of observations in different bands of the electromagnetic spectrum, from radio waves to gamma rays, we now know that GRBs are produced by jets of energy and matter launched from a black hole and expanding almost at the speed of light. The particles transported by the jet are accelerated through shock waves or in magnetic reconnection regions, converting the kinetic (or magnetic) energy into electromagnetic radiation, which typically peaks in gamma rays. Subsequently, the jet emits radiation at lower frequencies when it encounters and interacts with the interstellar medium, producing to the so-called afterglow phase, which can last from minutes, hours up to months after the initial burst. The steep decay represents the phase between the conclusion of the initial prompt phase, where most of the high-energy radiation is released, and the beginning of the afterglow. Its characteristic steepness of the flux decay is generally associated with the curved geometry of the jet surface inside which the particles are accelerated and then dissipate their energy in the form of radiation.
However, this scenario, long accepted by the scientific community to interpret the steep decay, fails to reproduce the spectral relation found by this new work. The observational evidence imposed a paradigm change for the theoretical interpretation. The solution to interpret the observations was found by assuming an adiabatic cooling of particles. By cooling we mean the process through which the particles lose their initial energy, while the term adiabatic refers to the expansion of the volume that contains the particles, which does not exchange heat with the external environment.
“I started to realize that we were observing something unique when in the data analysis I continued to find this strong spectral evolution, independent of the characteristics of gamma-ray bursts. Since that moment we began to understand the potential of the result “- says Samuele Ronchini, a third year PhD student at the GSSI and he adds “Questioning models well-rooted in the scientific community is certainly not easy and carrying out this work was a significant responsibility. However, the perseverance, competence and support of the entire research group made it possible to go all the way and obtain the desired results “.
This result has a deep impact on the understanding of the processes of emission and cooling of accelerated particles in GRBs.
“The dominance of the adiabatic process indicates that the particles are unable to efficiently dissipate their energy, giving valuable information on the physical properties of the relativistic jet, such as the evolution of the magnetic field in the acceleration site, as well as on the nature of the particles themselves” – explains Gor Oganesyan, post-doc at GSSI and second author of the article – “In particular, we understood that a mild decay of the magnetic field is necessary to reproduce the observed data, together with adiabatic cooling”.
Furthermore, the inefficient energy dissipation of the accelerated particles could suggest thar protons, and not electrons, emit radiation via the synchrotron process. Om Sharan Salafia, from INAF in Milan and co-author of the study, concludes: “GRBs are particularly difficult to study because, despite having some distinctive characteristics, they are extremely different from each other. Finding common and universal features, as in this case, is the key to unify them and understand the physical processes that produce them”.
Future wide-field X-ray observatories able to monitor the entire evolution of GRBs from the very first moments after the explosion will be crucial to fully understand the result found, by extending the work to a larger sample and in other bands of the spectrum. Marica Branchesi, full professor at the Gran Sasso Science Institute and co-author of the work, underlines: “Understanding the mechanisms at the basis of these catastrophic events is of primary importance also in the context of multi-messenger astronomy. The combined study of gravitational waves and electromagnetic radiation associated with GRBs are expected to clarify many open questions related to the nature of the compact objects that generate them, their distribution on cosmological scales, as well as the physics of jets and the acceleration of particles inside them.” The research leading to these results has received funding from the European Union’s Horizon 2020 Programme under the AHEAD2020 project (grant agreement n. 871158).
Link articolo Nature Communications https://www.nature.com/articles/s41467-021-24246-x
I getti dei lampi gamma si “raffreddano” così
Pubblicato su Nature Communications lo studio guidato da Samuele Ronchini, dottorando al GSSI e associato INFN, che pone nuova luce sui processi di raffreddamento nei getti dei Gamma-Ray Burst fornendo preziose informazioni sulle proprietà fisiche di questi enigmatici eventi
Quali sono i processi che determinano il raffreddamento delle particelle relativistiche nei lampi gamma, dando origine alla tipica diminuzione rapida del flusso che accompagna l’esplosione iniziale? A dare una convincente risposta a questa domanda arriva oggi il lavoro di un gruppo di ricercatori del Gran Sasso Science Institute (GSSI), in collaborazione con colleghi dell’Istituto Nazionale di Astrofisica (INAF) in cui viene presentata una relazione che accomuna diversi lampi gamma (o GRB, Gamma-Ray Burst in inglese) durante una fase di transizione del fenomeno denominata “steep decay” (decadimento rapido). L’unicità di tale relazione ha spinto il gruppo ad andare alla ricerca di un processo universale che potesse darne una corretta interpretazione. Lo studio è stato pubblicato oggi sulla rivista Nature Communications.
I lampi gamma sono considerati tra gli eventi più catastrofici ed energetici dell’Universo poiché possono rilasciare in poche frazioni di secondo l’intera energia emessa da una stella come il Sole durante tutta la sua vita.
La scoperta di supernove e di onde gravitazionali associate a lampi gamma ha confermato che alcuni di questi fenomeni sono il prodotto del collasso di una stella massiccia, e altri della fusione di due stelle di neutroni. Noti fin dagli anni ‘60, la loro natura è rimasta dibattuta fino all’avvento del Neil Gehrels “Swift” Observatory, un osservatorio in orbita dal 2004 dedicato alla rilevazione, localizzazione e caratterizzazione dei GRB. Dopo anni di osservazioni in diverse bande dello spettro elettromagnetico, dalle onde radio ai raggi gamma, oggi sappiamo che i GRB sono prodotti da getti di energia e materia lanciati da un buco nero che si espandono a velocità prossime a quelle della luce. Le particelle trasportate dal getto vengono accelerate attraverso onde d’urto o in regioni di riconnessione magnetica, convertendo l’energia cinetica (o magnetica) in radiazione elettromagnetica, che tipicamente ha il suo picco nei raggi gamma. Successivamente il getto emette radiazione a più basse frequenze nell’incontro e interazione con il mezzo interstellare, dando origine alla cosiddetta fase di afterglow, che può protrarsi da minuti, ore fino a mesi dopo il lampo gamma iniziale. Il decadimento rapido (steep decay) segna il limite di demarcazione tra la conclusione della fase iniziale impulsiva, dove viene rilasciata gran parte della radiazione di alta energia, e l’inizio dell’afterglow. La sua caratteristica rapidità di decadimento in flusso è generalmente associata alla geometria curva della superficie del getto al cui interno le particelle vengono accelerate per poi dissipare la propria energia sotto forma di radiazione.
Tuttavia, questo scenario, da tempo accettato dalla comunità scientifica per interpretare lo steep decay, fallisce nel riprodurre la relazione spettrale trovata dal team di ricerca. L’evidenza osservativa ha quindi imposto una modifica di paradigma per l’interpretazione teorica e la soluzione è stata trovata assumendo un raffreddamento adiabatico delle particelle. Per raffreddamento si intende il processo attraverso il quale le particelle perdono la loro energia iniziale, mentre il termine adiabatico si riferisce all’espansione del volume che contiene le particelle, il quale non scambia calore con l’ambiente esterno.
“Iniziai ad accorgermi che stavamo osservando qualcosa di unico quando nell’analisi dei dati continuavo a trovare questa forte evoluzione spettrale, indipendente dalle caratteristiche dei lampi gamma. Da lì abbiamo iniziato ad intuire la potenzialità del risultato” – afferma Samuele Ronchini, studente al terzo anno di dottorato presso il GSSI e aggiunge “Mettere in discussione modelli ben radicati nella comunità scientifica non è certo semplice e portare avanti questo lavoro è stata una responsabilità significativa. Tuttavia la perseveranza, la competenza e l’appoggio di tutto il gruppo di ricerca hanno permesso di arrivare fino in fondo e di ottenere i risultati sperati”.
Tale risultato ha un profondo impatto sulla comprensione dei processi di emissione e raffreddamento delle particelle accelerate nei GRB.
“La dominanza del processo adiabatico indica che le particelle non riescono a dissipare efficacemente la propria energia, dando preziose informazioni sulle proprietà fisiche del getto relativistico, come l’evoluzione del campo magnetico nel sito di accelerazione, nonché sulla natura delle stesse particelle” – spiega Gor Oganesyan, post-doc presso il GSSI e secondo autore dell’articolo – “In particolare, abbiamo capito che per riprodurre i dati osservati è necessario un blando decadimento del campo magnetico, assieme al raffreddamento adiabatico”.
Inoltre, la dissipazione inefficiente di energia delle particelle accelerate potrebbe suggerire che siano i protoni, e non gli elettroni, ad emettere radiazione tramite processo di sincrotrone. Om Sharan Salafia, dell’INAF a Milano e co-autore dello studio, conclude: “I GRB sono particolarmente difficili da studiare perché, nonostante abbiano alcune caratteristiche distintive, sono estremamente diversi l’uno dall’altro. Trovare dei tratti comuni e universali, come in questo caso, è la chiave per unificarli e capire i processi fisici che li producono”.
Futuri osservatori in banda X a largo campo di vista in grado di monitorare l’intera evoluzione dei GRB fin dai primi istanti dall’esplosione saranno cruciali per comprendere a fondo il risultato trovato estendendo il lavoro ad un campione più ampio e in altre bande dello spettro. Marica Branchesi, professore ordinario al Gran Sasso Science Institute e co-autrice del lavoro, sottolinea: “La comprensione dei meccanismi alla base di questi eventi catastrofici è di primaria importanza anche nel contesto dell’astronomia multimessaggera. Lo studio combinato di onde gravitazionali e radiazione elettromagnetica associate ai GRB potrà in futuro chiarire molte questioni aperte legate alla natura degli oggetti compatti che li generano, alla loro distribuzione su scale cosmologiche, nonché alla fisica dei getti e all’accelerazione di particelle al loro interno”.
La ricerca che ha portato a questi risultati ha ricevuto finanziamenti dal programma Horizon 2020 dell’Unione Europea nell’ambito del progetto AHEAD2020.