High-resolution X-ray spectrometers are revolutionizing our understanding of the Universe. These instruments allow scientists to uncover the secrets of galaxies, clusters of galaxies, and the behavior of matter near black holes. However, analyzing the data from these advanced instruments requires precise knowledge of atomic processes. That’s where Work Package 13 (WP13) of the AHEAD2020 project steps in. Here, we highlight WP13’s contributions to laboratory astrophysics and its critical role in supporting future space missions like Athena and XRISM.
What is WP13 All About?
WP13 focuses on laboratory astrophysics—conducting experiments on Earth to better understand atomic processes that occur in space. This knowledge helps scientists analyze the high-resolution X-ray spectra that future space telescopes will capture. The work addresses three main challenges:
- Improving Theoretical Models: Enhancing calculations of how atoms and ions interact and emit X-rays in extreme environments, such as those found in space.
- Laboratory Measurements: Performing precise experiments to measure X-ray wavelengths and cross sections of elements found in stars, galaxies, and beyond.
- Understanding Background Noise: Investigating and reducing particle-induced noise that can affect the data collected by space telescopes.
Key Results and Highlights
1. Better Atomic Data for X-ray Spectra
To improve our understanding of how elements emit X-rays, scientists conducted detailed laboratory measurements of highly charged ions (atoms missing many electrons). These experiments were performed using a cutting-edge X-ray detector, the SRON TES microcalorimeter, which was installed on the Flash Electron Beam Ion Trap (EBIT) at the Max Planck Institute for Nuclear Physics in Heidelberg (Figure 1). This setup allowed measurements with incredible precision, detecting X-ray features with an accuracy of 2-3 eV across the 0.3-10 keV energy range (Figure 2).
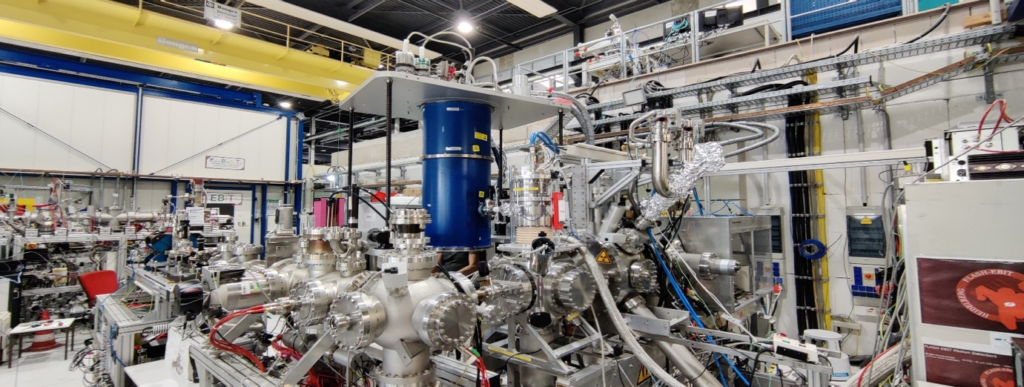
The experiments studied elements such as neon, chlorine, sulfur, argon, titanium, iron, and nickel, as well as processes like dielectronic recombination (a type of atomic interaction). These results provide vital data for refining theoretical models and better interpreting the X-ray spectra from space.
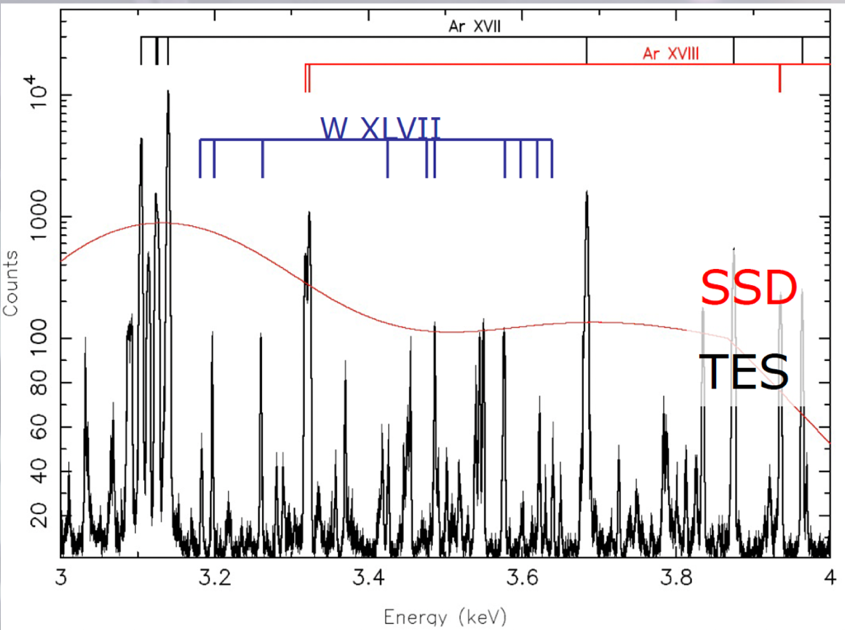
Figure 2: First light spectrum of TES-EBIT experiment. The spectrum obtained with a silicon drift detector (SSD) is shown in red, and the TES spectrum is shown in black. The positions of the He- Ar, H- Ar, and W47+ lines are marked.
2. Understanding Photoionization
Photoionization happens when high-energy light hits an atom, causing it to lose electrons. This process is common in space and influences the light we observe from stars and galaxies. To study this, WP13 scientists measured the energies and cross sections (a measure of the likelihood of interaction) for elements like nitrogen, oxygen, and carbon (Figure 3).
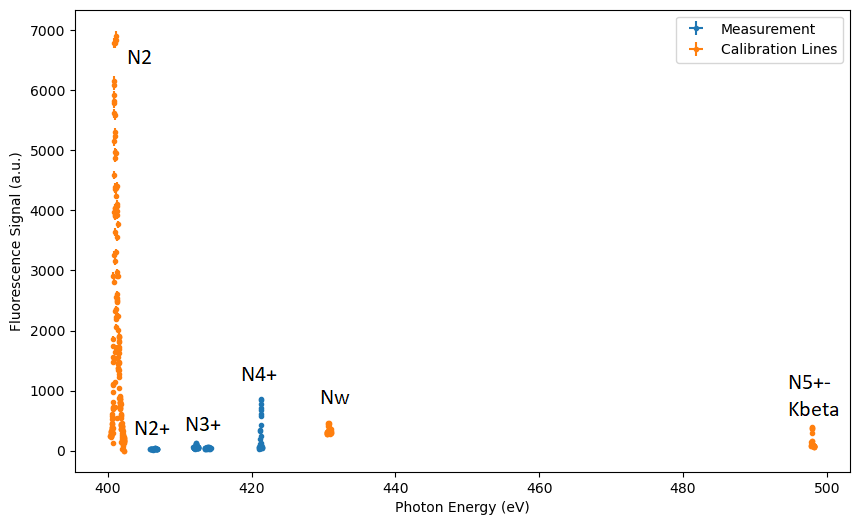
Figure 3: Photoionization spectra of N2+, N3+, N4+, the molecular N2, Nw, and N5+kbeta.
These experiments used advanced facilities, including the Elettra Synchrotron Laboratory and a compact EBIT. Scientists ensured accuracy by calibrating their measurements with molecular nitrogen and ions of nitrogen and carbon. These results improve our ability to model and understand astrophysical environments where photoionization occurs.
3. Reducing Background Noise for Athena
Space telescopes like Athena face challenges from unwanted background noise caused by particles in space. This noise can obscure the faint signals from distant cosmic objects. WP13 tackled this issue by studying how low-energy protons and secondary electrons interact with materials used in Athena’s mirrors.
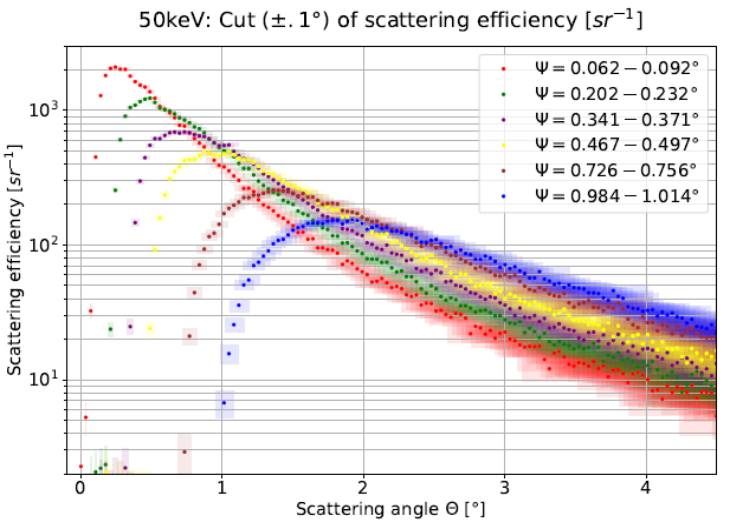
Experiments at the University of Tübingen tested how protons scatter and lose energy when hitting materials like Silicon Pore Optics (SPO) coated with thin layers of iridium and silicon carbide (Figure 4). These measurements help refine simulations predicting background noise, ensuring cleaner and more reliable data from future missions.
Why Does This Matter?
WP13’s work is essential for unlocking the full potential of next-generation X-ray telescopes. By providing accurate atomic data and addressing challenges like background noise, scientists can better interpret the signals from the most extreme and distant regions of the Universe. This research brings us closer to answering fundamental questions about the cosmos, from how galaxies form to what happens near black holes.